2 - Manipulation
2 - Manipulation
Control and manipulation of the pulse-mode structure of light in the classical domain often works on principles of amplitude filtering and gain, which do not preserve the fragile quantum features of non-classical states. For example, using a spectral filter to generate narrow bandwidth pulses from initially broader pulses works well with classical lasers, since photon statistics of laser light are robust to loss. However, generating a single-photon pulse with a narrowed spectrum from an initially broader single photon by spectral filtering does not work; it introduces vacuum and leads to a mixed output state. This example highlights the key characteristics required to manipulate the spectral-temporal mode structure of quantum states – lossless unitary transformations of the field. Here we are developing various approaches to coherently control the pulse-mode structure of light that preserve its quantum nature.
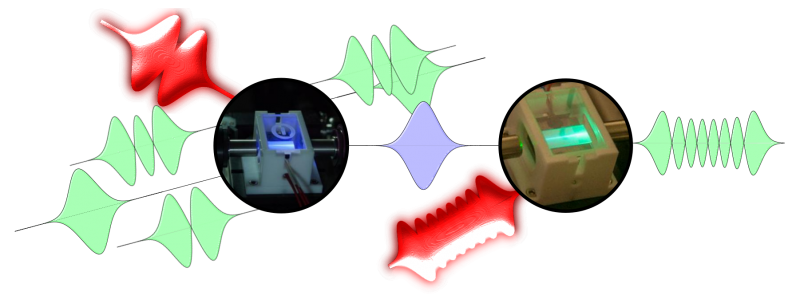
If you have questions, please feel free to contact John M. Donohue from the University of Paderborn.
Quantum memories

Quantum memories are first and foremost thought of as devices that store a quantum state of light, in our case a quantum rainbow, just as a classical memory stores classical information. It turns out however, that quantum memories can do more: they can actually store a specific quantum rainbow and return it in a different shape. This capability has many applications. You can use a quantum memory to spell a message, by imprinting “letters” on your quantum states. Alternatively, you could use them as a translator for different alphabets, allowing different devices to talk to each other.
The quantum memory we have developed during QCUMbER is based on a hot gas of Caesium atoms. This has several benefits: first, we do not need a complex setup (e.g. a “super fridge” or cryostat) to operate our memory; second, we can use broadband photons, which makes our memory compatible with high clock-rate networks; finally, we can operate our memory with simple lasers, allowing for a low-cost and compact packaging in the future.
There is still work to be done before our memory will be ready for commercialisation, but we have taken the important first step. We have demonstrated that our memory operates on only one user-chosen quantum rainbow and we have shown that we can interface different quantum rainbows. Currently, we are taking the next step by reducing the quantum noise level of our memory to a point where we can interface it with genuine single photons.
Question? Ask Benni Brecht from the University of Oxford.